Researchers at the University of Queensland's Australian Institute for Bioengineering and Nanotechnology (AIBN) have developed a new approach to delivering DNA into cells using spikey silica nanoparticles. These nanoparticles transport the genetic cargo across the cell wall while protecting it from degradation.
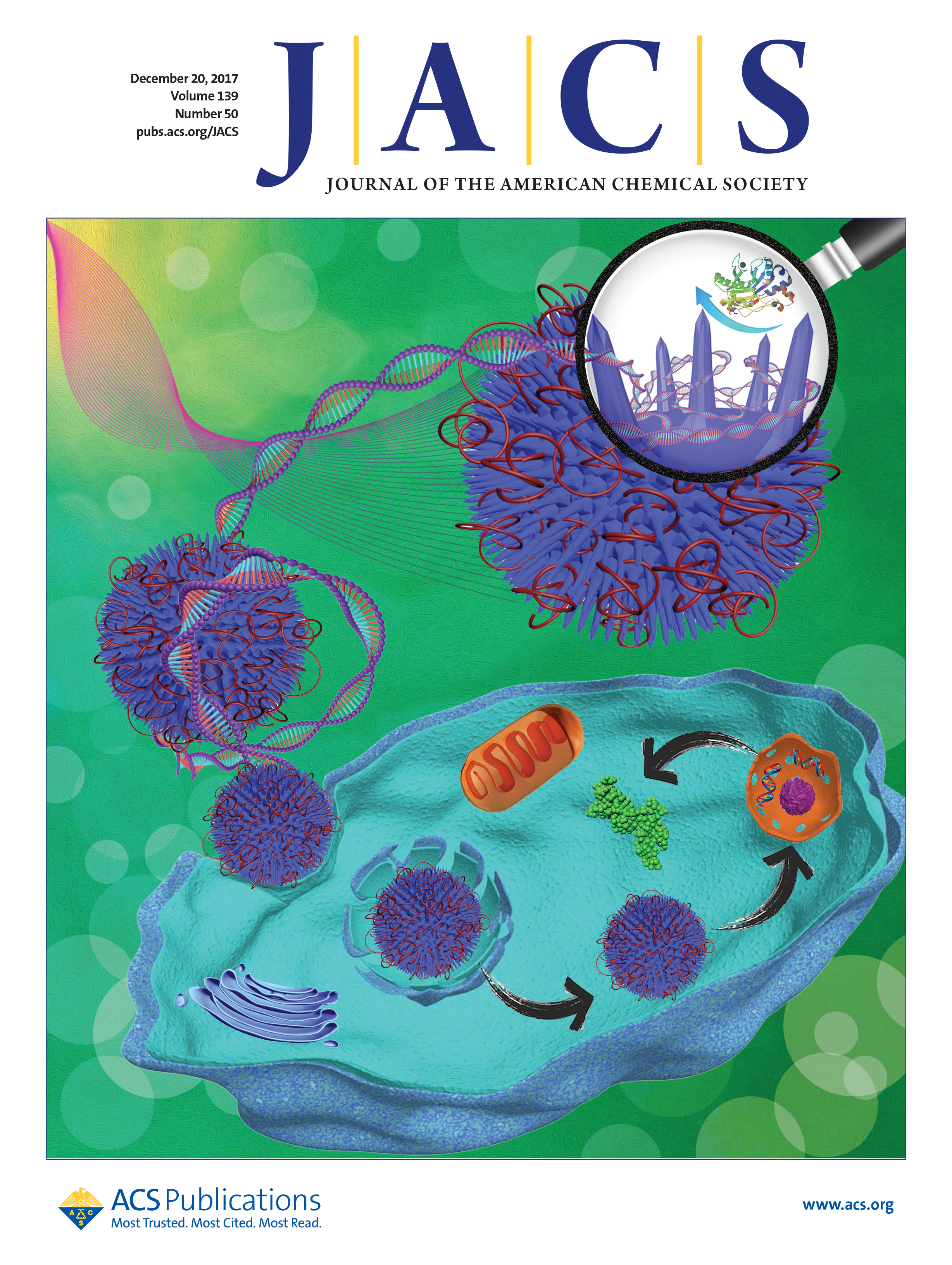
The findings were recently published in the Journal of the American Chemical Society (JACS).
The ability to safely and effectively deliver genetic information to cells has a range of potentially important applications. This is particularly the case in medicine where the use of DNA delivery in gene therapy and DNA vaccines could yield substantial health benefits.
Unfortunately there are a host of challenges involved in delivering DNA into cells.
AIBN Professor Michael Yu, who led the research, explains that DNA is particularly difficult to get across a cell membrane due to its size. Compared to other genetic molecules, DNA is the largest in terms of molecular weight. It is not going to be passively diffused into a cell, rather it requires a carrier.
Viral systems have traditionally been used, because delivering genetic material into a cell is what viruses are good at. Unfortunately, this approach can have toxicity issues or trigger immune reactions.
“Better strategies are needed,” says Yu.
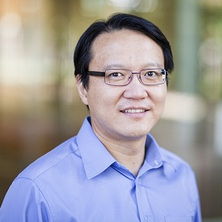
In order to develop a safe and effective transport mechanism, Yu and his research group, including PhD student Hao Song, turned their attention to silica nanoparticles.
Silica nanoparticles are known to be internalised by cells. They also have versatile surface chemistry meaning that a positively charged coating can be applied to the particle, which enables it to hold onto the negatively charged DNA. Moreover, over the past years the Yu research group has developed several unique techniques to tailor the exact three dimensional structure of the surface of silica nanoparticles.
The new research shows that this surface topography is extremely important in DNA delivery.
Yu and his colleagues created three surface designs: rambutan, raspberry, and flower-like.
The ‘rambutan’ shape is covered in silica spikes, named after the spine-covered fruit pods of the rambutan tree. In this design, the DNA remained mostly extended, weaving through the spikes.
The raspberry structure has small silica hemispheres all over the surface and the DNA weaves around them. Finally, the flower-like shape contains indentations, similar to bowls, which the DNA dips in and out of.
The researchers wanted to see which shape could bind DNA well and transport it into cells, particularly in the presence of degrading enzymes. This is important because, in the case of gene therapy, if the genetic material is broken down by enzymes in the body then the therapy won’t be effective.
The best of the three by far was the spikey shape. With this design, the DNA remains relatively extended and the spikes provide an extra surface area for interaction. Moreover, the spikes protect the DNA from degradation by physically preventing enzymes from reaching the DNA. Conversely the hemisphere design and the bowl design leave the DNA exposed to enzyme attack.
When the researchers compared the spikey nanoparticles with a commercial agent commonly used to transport DNA into cells the spikey nanoparticles were substantially better at getting DNA into cells in the presence of degrading enzymes.
“Ours provides much better protection,” Yu explains.
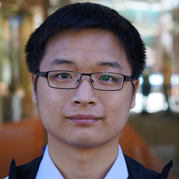
Hao Song points out that the silica is also very biocompatible. “It can be degraded in body fluid within a couple of days."
Importantly, they were able to show that the spikey nanoparticles were able to successfully deliver DNA into cells.
They loaded the nanoparticles with the gene for Green Fluorescent Protein and showed that the gene not only entered the cells, but also reached the nucleus where the genetic instructions were followed and Green Fluorescent Protein was subsequently produced. As a result, the cells glowed green in a fluorescence microscope.
“This is the first study to show how surface topography is important for binding and protecting DNA for delivery,” says Yu.
The new design has potential in gene therapy applications, and the technology has already been licensed to a commercial partner to develop a DNA vaccine.